Blogs
- Home
- Blogs
From Soil Testing to Slope Stability: Understanding the Fundamentals of Geotechnical Engineering
Protrainy | Aug. 4, 2023, 12:39 p.m.
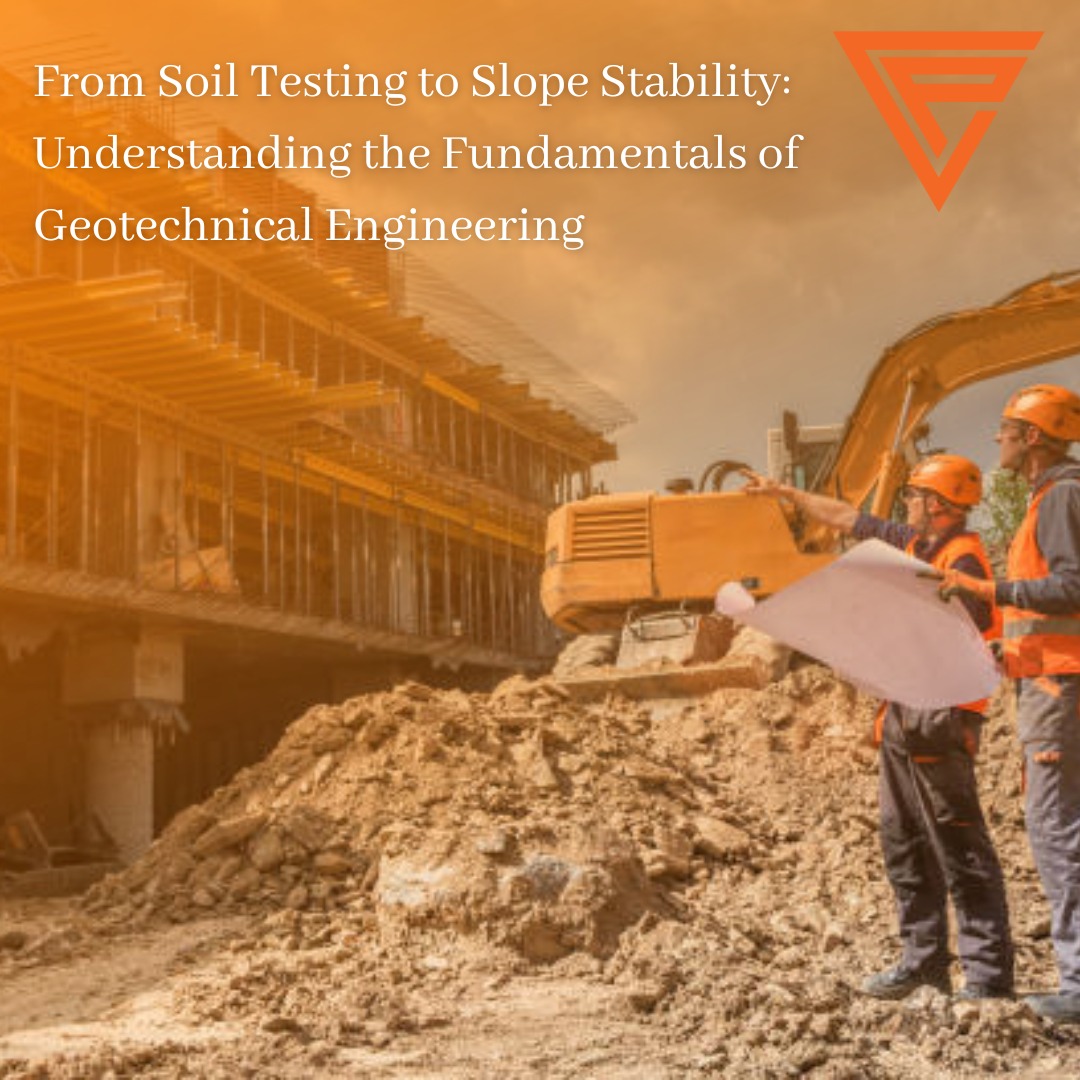
Welcome to the world of geotechnical engineering, where the ground beneath our feet holds the key to building a strong and stable foundation. Whether you're a construction professional looking to deepen your understanding or simply curious about the science behind soil testing and slope stability, this article is your gateway to the fundamentals of geotechnical engineering. In this comprehensive guide, we'll explore the crucial role of geotechnical engineering in construction projects, uncover the secrets behind soil testing techniques, and delve into the complexities of slope stability analysis. From the intricate details of soil composition to the importance of understanding geologic hazards, we'll equip you with the knowledge to make informed decisions and ensure the safety and longevity of any structure. So, let's dig in and discover the fascinating world of geotechnical engineering together. Importance of Soil Testing in Geotechnical Engineering Soil testing is a fundamental aspect of geotechnical engineering that plays a crucial role in the design and construction of any infrastructure. By understanding the properties and behavior of soil, engineers can assess its suitability for supporting structures such as buildings, bridges, and roads. Soil testing provides valuable information about the composition, strength, and permeability of the soil, allowing engineers to make informed decisions about foundation design, slope stability, and site suitability. It helps identify potential hazards such as expansive soils, liquefaction, or landslides, which can pose significant risks to the integrity of structures. Without proper soil testing, construction projects are vulnerable to costly failures and compromised safety. Soil testing involves a range of techniques and methods to collect soil samples and analyze their properties. These tests can be performed in a laboratory or in situ, depending on the requirements of the project. Standard penetration tests, cone penetration tests, and shear strength tests are some of the common soil testing methods employed in geotechnical engineering. Each test provides valuable insights into the soil's behavior under different conditions, enabling engineers to make accurate predictions and design structures that can withstand the forces exerted by the soil. Understanding the properties of soil is essential for effective geotechnical engineering. The strength and stiffness of the soil determine its load-bearing capacity, which directly affects the structural design and stability of any construction project. The permeability of the soil affects its ability to drain water, preventing the buildup of excess pore pressure and potential instability. By conducting comprehensive soil tests, engineers can gain a deeper understanding of these properties and make informed decisions about foundation design, slope stability, and site suitability. Soil testing is the backbone of geotechnical engineering, providing the necessary data for designing safe and resilient structures. Types of Soil Tests for Geotechnical Engineering In geotechnical engineering, various types of soil tests are conducted to assess the behavior and properties of soil. These tests help engineers determine the suitability of the soil for different construction projects and identify potential hazards that may affect the stability of structures. Let's explore some of the common soil tests used in geotechnical engineering. 1. **Standard Penetration Test (SPT):** The Standard Penetration Test is a widely used method for determining the relative density and strength of soil. It involves driving a split-barrel sampler into the ground and measuring the number of blows required to penetrate the soil to a specified depth. The SPT provides valuable information about the soil's resistance to penetration, which is used to assess its shear strength and compactness. This test is especially useful in determining the bearing capacity of the soil and designing foundations. 2. **Cone Penetration Test (CPT):** The Cone Penetration Test is another commonly used method for evaluating the properties of soil. It involves pushing a cone-shaped penetrometer into the ground and measuring the resistance encountered during penetration. The CPT provides information about the soil's frictional and cohesive properties, as well as its strength and compressibility. This test is particularly useful in assessing the liquefaction potential of soils, which is essential in seismic design. 3. **Shear Strength Test:** The Shear Strength Test is conducted to determine the soil's resistance to shear forces. It measures the maximum shear stress that the soil can sustain before failure occurs. This test helps engineers understand the stability of slopes and the potential for landslides. By analyzing the shear strength parameters, such as cohesion and angle of internal friction, engineers can design effective slope stabilization measures and ensure the safety of structures built on slopes. These are just a few examples of the many soil tests available in geotechnical engineering. Each test provides valuable information about the soil's properties and behavior, enabling engineers to make informed decisions about design and construction. By conducting a comprehensive suite of soil tests, engineers can gather a complete picture of the soil's characteristics and design structures that are safe, durable, and resilient. Understanding Soil Mechanics in Geotechnical Engineering Soil mechanics is a branch of geotechnical engineering that focuses on the behavior of soil and its interaction with structures. It involves the study of various properties of soil, such as its composition, density, strength, and permeability, and how these properties affect the stability and performance of structures. Understanding soil mechanics is essential for designing foundations, analyzing slope stability, and predicting the behavior of soil under different loading conditions. Soil is a complex material composed of solid particles, water, and air. The arrangement and characteristics of these components determine the engineering properties of the soil. The interparticle forces, such as friction and cohesion, play a significant role in the soil's strength and stability. The density and compaction of the soil affect its load-bearing capacity and ability to resist settlement. The presence of water in the soil affects its permeability and potential for consolidation. In geotechnical engineering, soil mechanics principles are used to analyze the behavior of soil and predict its response to different forces. By conducting laboratory tests and field investigations, engineers can determine the soil's properties and use this information to design safe and efficient foundations. Soil mechanics also helps engineers understand the causes of soil failures, such as landslides or settlements, and develop appropriate mitigation measures. The study of soil mechanics involves various concepts and theories, such as effective stress, consolidation, and shear strength. Effective stress is a fundamental principle that relates the stress applied to the soil to the soil's response. It accounts for the influence of pore water pressure on the soil's strength and stability. Consolidation is the process by which saturated soils gradually compress under an applied load, leading to settlement. By understanding the consolidation behavior of soil, engineers can predict the long-term settlement of structures and design appropriate foundation systems. Shear strength is another crucial concept in soil mechanics. It refers to the soil's resistance to shear forces and is determined by the frictional and cohesive properties of the soil. Shear strength is essential in analyzing slope stability, designing retaining structures, and assessing the safety of excavations. By applying the principles of soil mechanics, engineers can analyze and predict the behavior of soil under different conditions, ensuring the safety and stability of structures. Soil mechanics is the foundation of geotechnical engineering, providing the theoretical framework for understanding and solving complex soil-related problems. Factors Affecting Slope Stability in Geotechnical Engineering Slope stability is a critical aspect of geotechnical engineering that deals with the analysis and design of slopes to prevent landslides and ensure the safety of structures. Understanding the factors that affect slope stability is essential for engineers to design effective stabilization measures and mitigate the risks associated with unstable slopes. Let's explore some of the key factors that influence slope stability in geotechnical engineering. 1. **Slope Geometry:** The geometry of a slope, including its height, angle, and shape, has a significant influence on its stability. Steep slopes are inherently more prone to instability due to the increased gravitational forces acting on the soil. The angle of the slope affects the shear strength of the soil and its resistance to sliding. The shape of the slope can also impact stability, as irregular slopes may have localized areas of weakness or increased stress concentrations. 2. **Soil Properties:** The properties of the soil, such as its cohesion, angle of internal friction, and shear strength, play a crucial role in slope stability. Cohesion refers to the binding forces between soil particles and is particularly important in cohesive soils such as clay. The angle of internal friction represents the resistance to shear along the soil particles. Soils with high cohesion and friction angles are generally more stable than those with low values. The water content and permeability of the soil also affect slope stability, as saturated soils have reduced shear strength and are susceptible to landslides. 3. **Groundwater Conditions:** The presence and movement of groundwater in the slope can significantly affect its stability. Excess pore water pressure can reduce the effective stress in the soil, making it weaker and more susceptible to failure. Water can also increase the weight of the soil, exerting additional forces on the slope. Changes in groundwater levels due to rainfall or other factors can lead to slope instability, especially in areas with high water table or poor drainage. 4. **Slope Loading:** The loading on a slope, such as the weight of structures, vehicles, or additional soil, can influence its stability. The placement of heavy structures or concentrated loads on the slope can induce additional stresses and increase the likelihood of failure. Changes in loading conditions, such as construction activities or changes in land use, should be carefully considered to ensure slope stability. 5. **Vegetation and Erosion:** Vegetation plays a vital role in slope stability by providing root reinforcement and absorbing excess water. The presence of vegetation can increase the cohesion of the soil, reducing the risk of landslides. On the other hand, the removal of vegetation or erosion of soil can weaken the slope and make it more susceptible to failure. Changes in the vegetation cover or erosion patterns should be monitored and managed to maintain slope stability. By considering these factors and conducting detailed slope stability analyses, engineers can design effective stabilization measures and ensure the safety of structures built on slopes. Slope stability is a complex and multidisciplinary field that requires a thorough understanding of geotechnical engineering principles and soil mechanics. Methods for Slope Stability Analysis The analysis of slope stability is a critical component of geotechnical engineering that involves assessing the safety and stability of slopes to prevent landslides and protect structures. Engineers employ various methods to analyze slope stability, ranging from simple analytical approaches to complex numerical modeling techniques. Let's explore some of the commonly used methods for slope stability analysis. 1. **Limit Equilibrium Analysis:** Limit equilibrium analysis is a widely used method for assessing slope stability. It is based on the principle of equilibrium, which states that the forces acting on a slope must balance to prevent failure. The method involves dividing the slope into slices and calculating the forces and moments acting on each slice. By comparing the driving forces (such as gravitational forces) with the resisting forces (such as shear strength), engineers can determine the factor of safety, which indicates the slope's stability. This method is relatively simple and provides a quick assessment of slope stability. 2. **Finite Element Analysis (FEA):** Finite element analysis is a numerical modeling technique used to simulate the behavior of complex structures and soil systems. In slope stability analysis, FEA involves discretizing the slope into finite elements and solving the governing equations of soil mechanics. This method can account for non-linear soil behavior, complex geometry, and boundary conditions, providing a more accurate representation of the slope's response. FEA requires advanced software and expertise in numerical modeling but offers a higher level of accuracy and flexibility compared to other methods. 3. **Slope Stability Charts:** Slope stability charts, also known as nomograms or graphical methods, provide a simplified approach to slope stability analysis. These charts are based on empirical relationships between slope geometry, soil properties, and stability conditions. By plotting the relevant parameters on the chart, engineers can determine the factor of safety and assess the slope's stability. Slope stability charts are particularly useful for preliminary assessments and quick estimations but may have limitations in representing complex slope conditions. 4. **Probabilistic Methods:** Probabilistic methods involve assessing slope stability in terms of probabilities and risk. These methods take into account the uncertainty and variability of soil properties and loading conditions, providing a more comprehensive assessment of slope stability. Probabilistic methods involve statistical analyses, such as Monte Carlo simulations or reliability analyses, to estimate the probability of failure and the associated risks. By considering the range of potential scenarios, engineers can make informed decisions about slope stabilization measures and risk mitigation strategies. These are just a few examples of the methods used in slope stability analysis. Each method has its advantages and limitations, and the choice of method depends on factors such as the complexity of the slope, available data, and project requirements. Regardless of the method employed, slope stability analysis is crucial for ensuring the safety and longevity of structures built on slopes. Geotechnical Engineering in Construction Projects Geotechnical engineering plays a vital role in construction projects by providing the necessary knowledge and expertise to ensure the safety and stability of structures. From the initial site investigation to the design and construction phases, geotechnical engineers are involved in every stage of the project. Let's explore the key aspects of geotechnical engineering in construction projects. 1. **Site Investigation:** Site investigation is the first step in any construction project and involves gathering information about the soil, rock, and groundwater conditions at the site. Geotechnical engineers conduct various tests and investigations, such as soil sampling, geophysical surveys, and groundwater monitoring, to understand the site's geologic and geotechnical properties. This information is used to assess the site's suitability for construction, identify potential hazards, and develop appropriate design and construction strategies. 2. **Foundation Design:** Foundation design is a critical aspect of geotechnical engineering that involves determining the type, size, and depth of the foundation to support the structure. Geotechnical engineers analyze the soil properties, load requirements, and stability conditions to design foundations that can safely transfer the loads to the ground. Various foundation types, such as shallow foundations, deep foundations, or pile foundations, may be used depending on the site conditions and structural requirements. The design of foundations takes into account factors such as bearing capacity, settlement, and lateral stability. 3. **Ground Improvement:** In some cases, the natural soil conditions may not be suitable for supporting the structure, requiring ground improvement techniques. Geotechnical engineers employ various methods to improve the soil's properties, such as compaction, soil stabilization, or soil reinforcement. Ground improvement techniques help increase the soil's strength, reduce settlement, and enhance its load-bearing capacity. These techniques are particularly useful in areas with soft or weak soils or when dealing with challenging site conditions. 4. **Slope Stabilization:** Slope stability is a